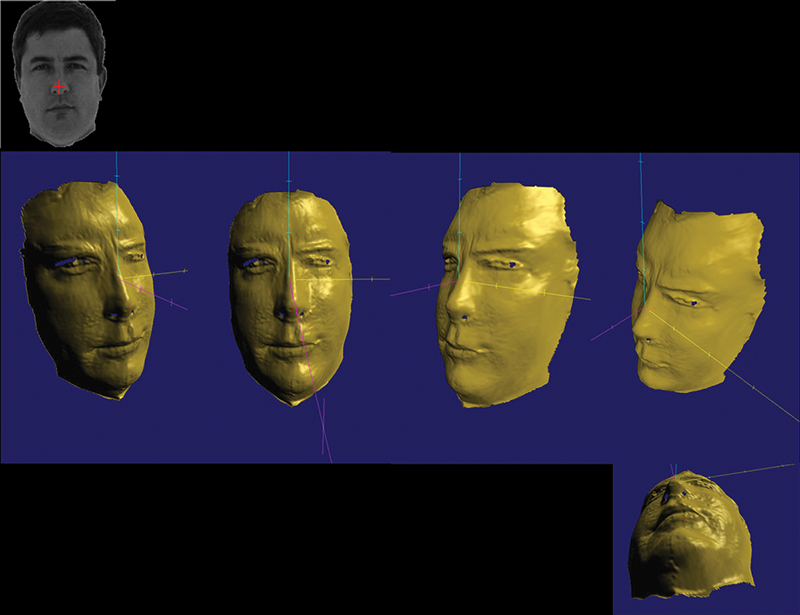
Spatial Phase Imaging
Originating Technology/NASA Contribution
In 1928, Alexander Fleming, a young Scottish scientist with a side practice of discretely treating the syphilis infections of prominent Londoners, was researching agents that could be used to combat such bacterial infections. He left his practice for a 2-week vacation, inadvertently leaving several bacterial culture plates unwashed and out of the incubator. When he returned, what immediately struck him was that the plates had grown mold, but the bacteria Fleming had been working with was being fended off by the mold, which he called penicillin, after the mold Penicillium notatum. Although unable to refine or purify the penicillin, Fleming had discovered the archetype of modern antibiotics.
The days of chance drug discovery and extensive trial-and-error testing are over, though. Drugs are not really discovered in this fashion anymore; rather, they are now designed.
Understanding proteins, the basic biological building blocks for all animals (including humans) and the regulators of biochemical processes in plants, helps researchers design these new drugs, combat diseases, and even improve agricultural products, such as pesticides. Researchers are unlocking this knowledge by studying the growth of protein crystals.
Through such study, researchers can now target a specific protein of a pathogen to maximize a drug’s effectiveness, while at the same time work to minimize possible side effects. This process, known as rational drug design, has one major downside: The exact structure of the target protein must be determined, down to the last molecule.
To uncover this molecular structure, scientists often use X-ray crystallography. A crystal of the protein is bombarded with X-rays to produce a pattern, which, much like a fingerprint, reveals the identity of the protein’s atomic structure. To get an accurate pattern, though, the crystal must be as free of imperfections as possible. Growing such crystals can be extremely difficult—even impossible—on Earth, because gravity causes the crystals to settle on top of one another, which results in structural flaws.
Since 1985, to take advantage of the ability of crystals to grow free of imperfections in microgravity, a variety of protein crystal growth experiments have flown on the space shuttle, and several have flown on the International Space Station (ISS).
Data collected from the ISS experiments allowed the comparison of growth rates and crystal quality of microgravity versus Earth-grown crystals. The crystals that are grown in microgravity are able to grow larger and better organized than on Earth. The research that is done on these crystals may further human space exploration efforts by technological and biological advancements developed as a direct result from this research and will likely lead to the newest generations of wonder drugs.
Partnership
Frequently, scientists grow crystals by dissolving a protein in a specific liquid solution, and then allowing that solution to evaporate. The methods used next have been, variously, invasive (adding a dye that is absorbed by the protein), destructive (crushing protein/salt-crystal mixtures and observing differences between the crushing of salt and protein), or costly and time-consuming (X-ray crystallography).
In contrast to these methods, a new technology for monitoring protein growth, developed in part through NASA Small Business Innovation Research (SBIR) funding from Marshall Space Flight Center, is noninvasive, nondestructive, rapid, and more cost effective than X-ray analysis. The partner for this SBIR, Photon-X, Inc., of Huntsville, Alabama, developed spatial phase imaging technology that can monitor crystal growth in real time and in an automated mode.
Spatial phase imaging scans for flaws quickly and produces a 3-D structured image of a crystal, showing volumetric growth analysis for future automated growth. It can measure the characteristics of a crystal and the crystal’s 3-D volumetric properties, and can also discriminate between salt and protein crystals.
The spatial phase imaging involves the use of proprietary filters. The operator uses a single camera to acquire a series of spatial phase images of a specimen—which could include one or more protein crystals mixed with one or more salt crystals. The next step is to digitally process the image data using algorithms that extract information on the 3-D properties of the protein crystal of interest, including its volume and some aspects of its crystalline structure. This information can be processed further to extract information about the symmetry of the crystal and to detect flaws
The method is not expected to eliminate the need for X-ray crystallography at the later stages of research. However, as a means of identification and preliminary analysis of protein crystals, it could eliminate or greatly reduce the need for X-ray crystallography as a screening technique in the early stages. In addition to being noninvasive and nondestructive, the new method yields results so rapidly that it is suitable for real-time monitoring and, hence, for providing process control feedback. This method is expected to accelerate the search for conditions to optimize the growth of proteins and to be a means of automation of the growth of high-quality protein crystals.
Product Outcome
While the target market for Photon-X’s spatial phase imaging technology includes pharmaceutical companies, as well as laboratories at the academic, commercial, structural, and governmental levels, this technology is very desirable to anyone who routinely sets up hundreds or thousands of crystallization experiments on a daily basis, and it is more cost effective than X-ray analysis.
Photon-X has also used this innovative technology to develop commercial 3-D cameras for various machine vision and automated 3-D vision systems. Its Spatial Phase Video Camera is able to extract 3-D information passively without scanned or structured lighting. This technology requires a single camera, a single-image capture, and is independent of range. The 3-D output is smooth and connected, with versatility and depth precision often exceeding that of triangulation-based methods. Plus, by eliminating the need for multiple camera angles, laser illumination, or moving targets to generate usable data, machine vision systems based on the sensor technology can be less expensive and easier to install, and as passive devices, present no laser radiation hazard to personnel.
The Photon-X 3-D spatial phase imaging system can rapidly provide highly accurate data about the surface features of its target. This information can be processed by existing applications to determine the area, volume, or height of surface features. This patented, innovative approach to characterizing surface elements is angle-invariant and easily scaled to suit a variety of applications, meaning that surface features can be recognized at a variety of distances and angles.
By simply changing its field of view, the technology has been successfully demonstrated in systems whose imaged targets range in size from microscopic crystals, to faces, automobiles, and aircraft; even to terrain features measuring hundreds of yards in width at distances up to several miles from the sensor unit itself.
Specific applications include machine vision, many different types of inspection, rapid prototyping, target or object recognition, surface damage assessments, deformation analysis, defect detection and characterization, terrain mapping, and biometric facial recognition, which analyzes a person’s facial characteristics through digital video input. The images can be used to construct intricate composites, which are then stored in a database. This method of identification is currently being used for security systems, but law enforcement agencies are now exploring its application in terrorist and criminal recognition.
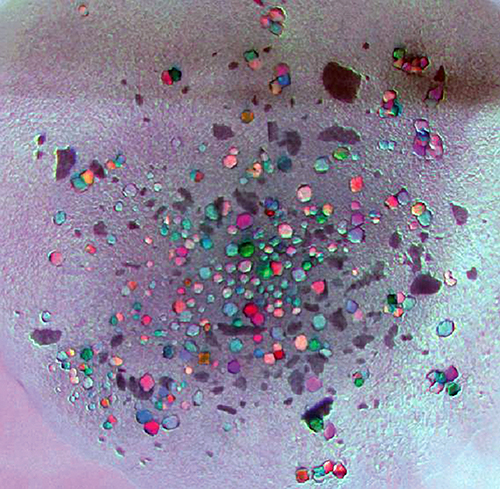
Crystallization trial of the glucose isomerase enzyme pseudo-colored by the phase of light from a slurry/crystal mixture and captured in 3-D.
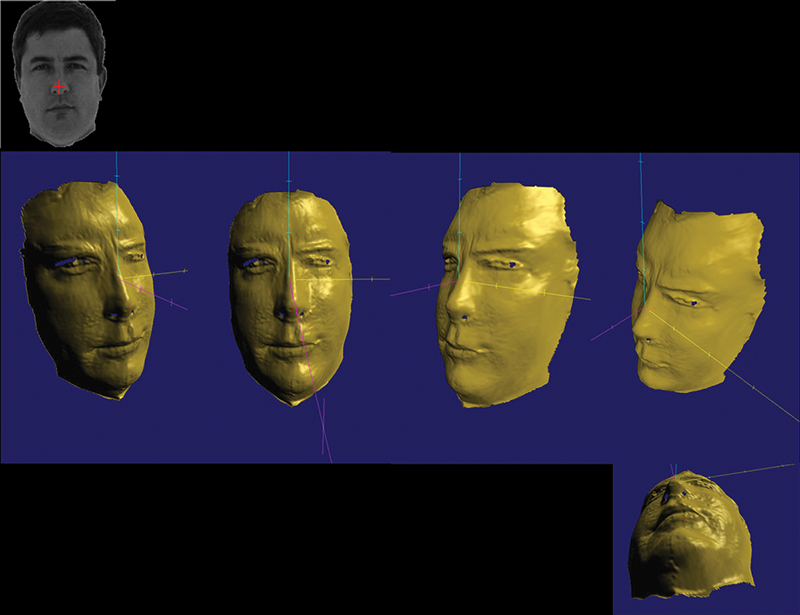
Photon-X’s 3-D camera technology can capture a 3-D image in single camera frame time. One application, face verification software, has been successfully demonstrated and shown to be a strong 3-D face verification tool.