Thermoelectric Devices Cool, Power Electronics
Originating Technology/NASA Contribution
More than 10 billion miles away from Earth, a NASA spacecraft continues a journey that began in 1977. Having long since accomplished its original mission to Jupiter and Saturn, Voyager 1 is the farthest human-made object from Earth, hurtling at more than 38,000 miles per hour toward the heliopause—the very edge of the solar system.
At these extraordinary distances, the Sun’s light is too faint to power a solar panel-equipped spacecraft. In fact, the Sun does not provide a viable source of power for many NASA missions. To accommodate this need, NASA employs thermoelectric (TE) devices, which can generate electricity from temperature differentials and vice versa. In a power-generating capacity, TE devices work via the Seebeck effect, in which a circuit made from dissimilar metals creates a voltage if a temperature difference exists between its two sides. When a voltage is applied to a TE device and a current flows through it, the reverse action occurs: The electrical input creates a temperature difference, moving heat from one side of the device to the other in what is called the Peltier effect. When functioning this way, TE devices become temperature management tools, cooling or heating a surface depending on the direction of the electrical flow.
For 25 missions so far—including Apollo missions to the Moon, the Viking and Pathfinder missions to Mars, and the Voyager, Pioneer, Ulysses, Galileo, and Cassini solar system missions—radioisotope thermoelectric generators (RTGs) have powered NASA spacecraft. A solid-state technology featuring no moving parts and thus significantly reducing the possibility of failure, these TE devices provide steady, reliable supplies of power for as long as their fuel emits enough heat; powered by three RTGs each, both Voyager 1 and its sister Voyager 2 (launched the same year) are expected to continue operation until 2025, a nearly 50-year lifespan.
Engineers at NASA’s Jet Propulsion Laboratory (JPL), who built and operate the Voyager spacecraft among other deep-space missions, continue to explore the potential of thermoelectrics for providing energy and thermal management solutions for use in space. Thermoelectrics have a host of applications on Earth as well, among them semiconductor electronics, lasers, infrared sensors, air conditioners, and communication systems. In keeping with the Agency’s mission to facilitate the transfer of space technology for public benefit, JPL has partnered with a North Carolina company to share the fruits of its research.
Partnership
In 2005, Dr. Jesko von Windheim and his team founded Nextreme Thermal Solutions Inc., based in Research Triangle Park, North Carolina, to commercialize thermoelectric technology acquired from RTI International, also headquartered in Research Triangle Park. Having previously licensed technology from the California Institute of Technology (Caltech) with microelectromechanical systems startup Cronos Integrated Microsystems, von Windheim was aware of JPL’s thermoelectric expertise and its expansive patent portfolio (Caltech manages JPL for NASA). He discovered a broad JPL thermoelectrics patent that complemented Nextreme’s RTI technology. In 2006, the company exclusively licensed the Caltech/JPL patent, which covers thermoelectric devices containing thermoelectric material less than 40 microns thick. The licensed technology enabled Nextreme’s development as a pioneer in solid-state thermal management for electronics and semiconductors.
“This license puts us in a strong position competitively in thin film thermoelectrics,” says Dr. Paul Magill, Nextreme’s vice president of marketing and business development.
Product Outcome
As electronics advance, becoming faster and more powerful while at the same time smaller and more densely built, engineers are running up against a significant challenge: heat. The lack of corresponding advances in cooling techniques has become a barrier to further development in integrated circuit technologies like microprocessors, where heat buildup can affect performance and lead to device failure. The heat generated by microchips is focused in hotspots rather than uniformly distributed, making conventional thermoelectric coolers (TECs)—also referred to as bulk modules and assembled from tiny pillars of thermoelectric material—impractical options due to their relatively large size. According to Nextreme, using system cooling options like fans, heat sinks, and refrigeration to address microchip hotspots is like air conditioning an entire house just to cool an overheated element on a kitchen stove. Nextreme’s licensed NASA technology is enabling the ideal solution for this problem: thin film thermoelectrics.
“We believe that the JPL technology is fundamental to thin film thermoelectric devices,” says von Windheim, Nextreme’s CEO. “We currently implement it in all of our products.”
Thin film thermoelectrics is exactly what it sounds like: ultra-thin thermoelectric technology. Using a process called metal-organic chemical vapor deposition, Nextreme engineers grow films of bismuth telluride which are then used to create the company’s breakthrough thermoelectric application: the thermal copper pillar bump.
Copper pillar bumps (CPBs) are solder bumps used to create mechanical and electrical connections as part of the flip chip method for joining semiconductor devices like integrated circuits to external circuitry. Nextreme adds thermal management capabilities to these bumps by integrating a thin film layer of thermoelectric material into each bump, converting them into TECs. As electricity is passed through the thermal bump, heat is moved through the Peltier effect from one side of the bump to the other, shifting it out of the chip and resulting in 5–15 °C of spot cooling per bump.
Using a modified CPB flip chip manufacturing process employed by major producers like Intel, Amkor, and Casio, designers can either uniformly distribute these thermal bumps (each 238 microns in diameter and 60 microns high) on a chip or locate them at specific hotspots for targeted thermal control. Nextreme notes that this implementation of thin film thermoelectrics is ideal considering that the flip chip market is one of the largest, fastest-growing semiconductor industry segments, and that as much as 40 percent of flip chip devices have significant thermal challenges. By reversing the current flow in a thermal bump, the device can switch between cooling and heating, providing precise, integrated thermal control.
“Now you can design new kinds of circuits that cool themselves, temperature stabilize themselves as a function of the circuit, not as an external cooling or refrigeration function,” says von Windheim. In a separate capacity, through the Seebeck effect, heat applied to one side of the thermal bump results in an electrical output—up to 10 milliwatts (mW) per bump, transforming the device into an embedded thermoelectric generator (eTEG).
The NASA-enabled thermal bump technology also forms the core of its discrete thermoelectric products, each of which is no larger than a piece of confetti. The OptoCooler product line is ideal for applications including photonics, optoelectronics like laser diodes and high-brightness light-emitting diodes (LEDs), and biomedical devices. On the energy generation side, Nextreme’s eTEG can produce up to 260 mW of power, providing a scalable and cost-effective technology for applications like medical implants and wireless sensor networks, the company says.
Nextreme continues to develop and improve its thin film thermoelectrics while expanding the technology’s possible uses to applications beyond electronics—such as cooling the human body. “The beauty of these products being so small,” says Magill, “is that they can be easily integrated into clothing and military body armor at places where the blood comes close to the skin” like the wrists or temples. As the body’s entire supply of blood is continuously cycled, the micro-TECs remove small amounts of heat, supplementing the body’s existing thermal management system and helping it cool.
The NASA technology developed to help power unprecedented journeys to the stars could also one day help people travel more effectively on Earth. The future could see Nextreme’s thermoelectric devices producing energy by harvesting normally wasted heat from engines, thus improving automobile fuel efficiency.
OptoCooler™ and eTEG™ are trademarks of Nextreme Thermal Solutions Inc.
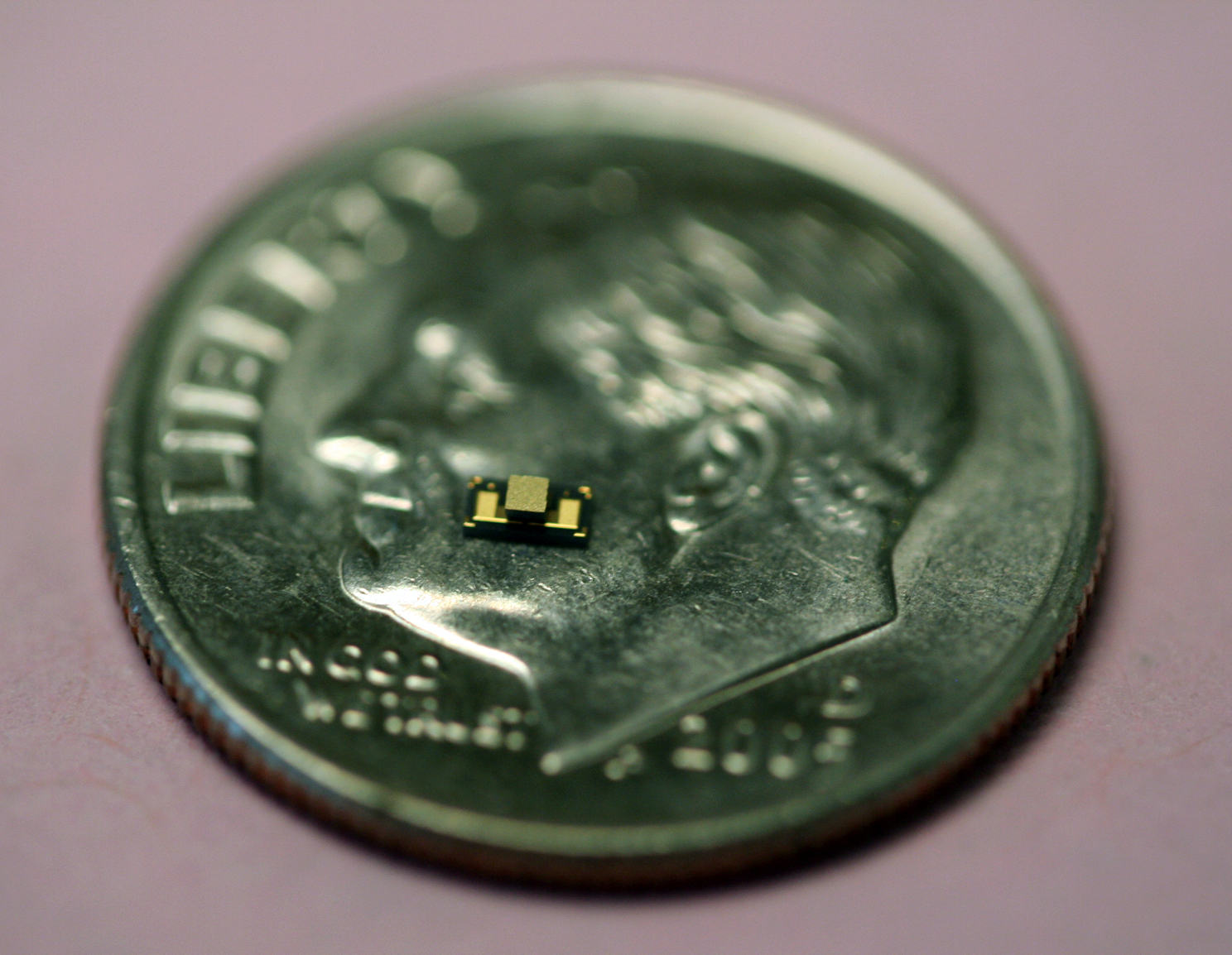
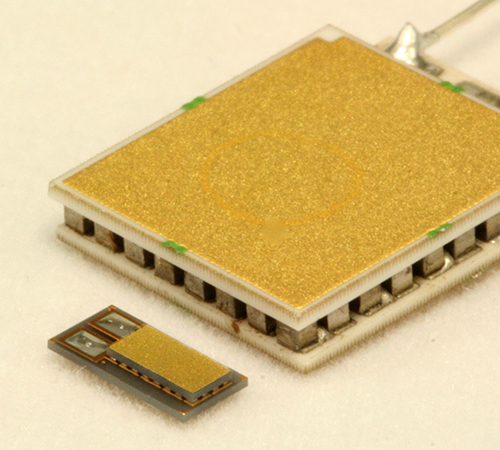
The small size of Nextreme’s thermoelectric coolers (top on dime, and in comparison to a conventional bulk module, bottom) is part of what makes them effective solutions for microchip hotspot cooling.
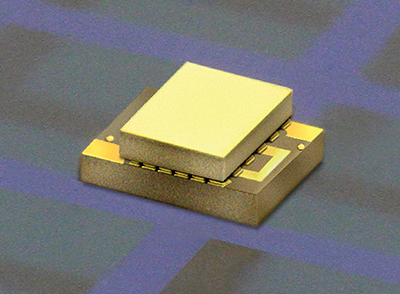
The company’s OptoCooler products, including this HV14 module, are enabled by thin film thermoelectric technology licensed from NASA.