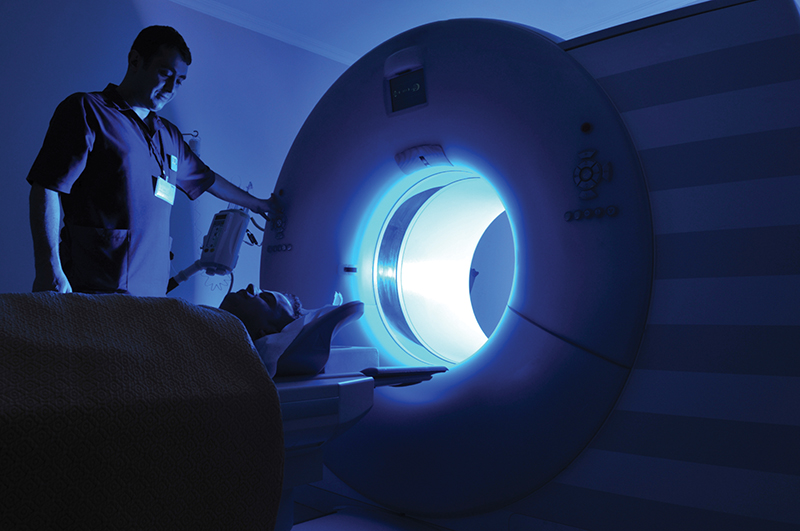
High-Temperature Superconductors Deliver Power without Heat
NASA Technology
It came to be known as the “Woodstock of Physics.” Thousands of scientists tried to squeeze into the Sutton Ballroom of the New York Hilton, overflowing into the aisles and halls, as the American Physical Society’s March 1987 meeting was about to get underway. The presentations would last until after three o’clock in the morning, with hundreds of attendees lingering until dawn, buzzing with excitement at the impending, revolutionary technological breakthroughs on the horizon.
Newspapers that year foretold electric cars, magnetically levitating high-speed trains, nuclear fusion plants, and extraordinary savings in energy. The miracle behind all the excitement? High-temperature superconductive (HTS) ceramics.
“At the time, there was great optimism that this would have huge commercial benefits,” says Ed Canavan, cryogenic engineer at NASA’s Goddard Space Flight Center. “It’s only now, almost three decades later, that we’re starting to get some of the HTS material into practical applications.”
Superconductive materials at very low temperatures allow electricity to pass with no resistance, zero losses, and maximum efficiency. Until the 1980s, the problem was that the amount of energy it took to attain the low temperatures that cause this phase change was often too large to realize the benefit of lower electrical losses. Superconducting metals require temperatures below about 20 K, or -424 °F. The high-temperature superconducting ceramics discovered in 1986 and ’87, on the other hand, undergo phase change at temperatures as high as 92 K (-294 °F).
“It’s not high-temperature in the normal sense of the term,” Canavan clarifies. But it’s a warm enough temperature to be maintained by liquid nitrogen, which boils at 77 K and can be bought for less than the price of bottled water or even produced onsite.
But these superconducting ceramics posed a host of technical challenges. They are chemically complex, and many are brittle and difficult to manufacture in useful lengths. Most challenging, the boundaries between their grains, if not perfectly aligned, cause steep reductions in the maximum current they can carry before losing superconductivity.
By the mid-2000s, the superconductor industry was able to move high currents over long distances using these ceramics arranged in a tape-like geometry, although the technology still was generally not cost-competitive.
In 2008, as work was getting underway on Japan’s ASTRO-H orbiting observatory, Canavan and other researchers at Goddard needed high-temperature superconducting leads to carry a current from a room-temperature power source to a cooling system operating near absolute zero. NASA is contributing the X-ray satellite’s high-resolution soft X-ray spectrometer, whose detectors are made ultrasensitive by temperatures about one-twentieth of a degree above absolute zero.
For its contributions to two previous, similar Japanese missions, Goddard had used delicate rods of the ceramic yttrium barium copper oxide (YBCO).
“If we had used normal, metallic wire, the heat carried by the wire from the middle-level temperature stage in the instrument to the lower-temperature region would just swamp the cooling power,” Canavan says. Metallic wire would introduce heat both by conducting it along its length and by generating heat as the electric current passed through it. A superconducting ceramic, on the other hand, is a poor conductor of heat and a perfect conductor of electricity, so it transmits little heat and produces none.
By the time work on ASTRO-H started, though, the company that had supplied the fragile YBCO rods no longer manufactured them. “In the meantime, Tai-Yang had developed a means for slitting superconducting tapes developed for electric power distribution down to a size reasonable for our application,” Canavan says, referring to the Knoxville, Tennessee-based company now known as Energy to Power Solutions, or E2P.
Technology Transfer
The company, which specializes in superconductivity and cryogenics, also made its superconducting tapes with YBCO, but they were much more flexible and durable than the cylinders NASA had used on previous missions.
“The challenge was that the allowable heat load was so small it was very difficult to come up with a design,” says Chris Rey, president of Energy to Power Solutions, noting that only 100 to 200 microwatts of heat could be transferred by the leads, which also had to be as compact as possible.
While mechanically more robust than solid ceramics, the micron-thick YBCO layer in these tapes is still sensitive to many factors, Canavan says. “It took a thorough knowledge of the material properties to be able to produce tapes small enough to meet the thermal requirements yet still capable of surviving all the processing and testing a flight component receives.”
The leads E2P delivered have a hybrid design, with normal metal wires carrying the current from the power source into the instrument and down to an intermediate-temperature connector. From this point, the superconducting leads carry the current down to a liquid helium tank, which operates at 1.2 K.
There it powers a superconducting electromagnet, Canavan explains. Inside the bore of the magnet is a cylinder of paramagnetic salt that heats up and cools down as the magnetic field is increased and decreased. By flipping heat switches that connect it to the liquid helium tank and the X-ray detector as the magnetic field ramps up and down, heat is drawn out of the detector at 0.05 K, lifted up to 1.2 K, and dumped into the helium tank.
NASA delivered the leads to Japan in July of 2014, where the spectrometer underwent months of testing. The satellite was scheduled to launch in late 2015.
Benefits
“In the world of astrophysics, there are a number of reasons that cooling your telescope and/or your detector is necessary to get the extreme sensitivity needed to see very faint, distant objects,” Canavan says. “Having these HTS leads that allow you to carry amps of current while not leaking heat down to your low-temperature region is a big advantage.”
While his company’s technology will help the ASTRO-H mission trace the growth of the universe’s largest structures, determine the spin of black holes and the properties of neutron stars, and examine the behavior of material in extreme gravitational fields, among other groundbreaking work, Rey is trying to put it to work here on Earth.
“We have had some commercial sales, but we would very much like to expand our commercial footprint,” he says, adding that, while other companies have similar technology, they have not developed the sort of ultra-low-heat lead his company created for NASA. One obvious market, he says, is in magnetic resonance imaging machines, which also use cryogenic superconductors to power their imaging technology.
“In general, superconductors are very useful devices for any time large amounts of power need to be conveyed in a small space,” he says.
Rey says the early expectations raised for high-temperature superconductive ceramics may have been unrealistic in terms of the time it would take to widely apply the technology (a 40-year timeline might have been more accurate), but not necessarily in the scope of the discovery’s impact. He says great progress has been made since the initial discovery. The materials’ complex chemistry posed a major challenge at first, followed by the difficulty of growing kilometer-length ceramic crystals with near-perfect grain alignment, another hurdle that has been cleared. Now, he says, the main obstacle is cost.
“To move to larger, more high-volume applications, you need to get through the cost-reduction stage that the niche applications provide,” Rey says—niche applications like the ones he’s working on, for use in space and on Earth. “What we have attempted to do is engineer novel, lower-cost solutions rather than the brute-force approach that others are attempting.”
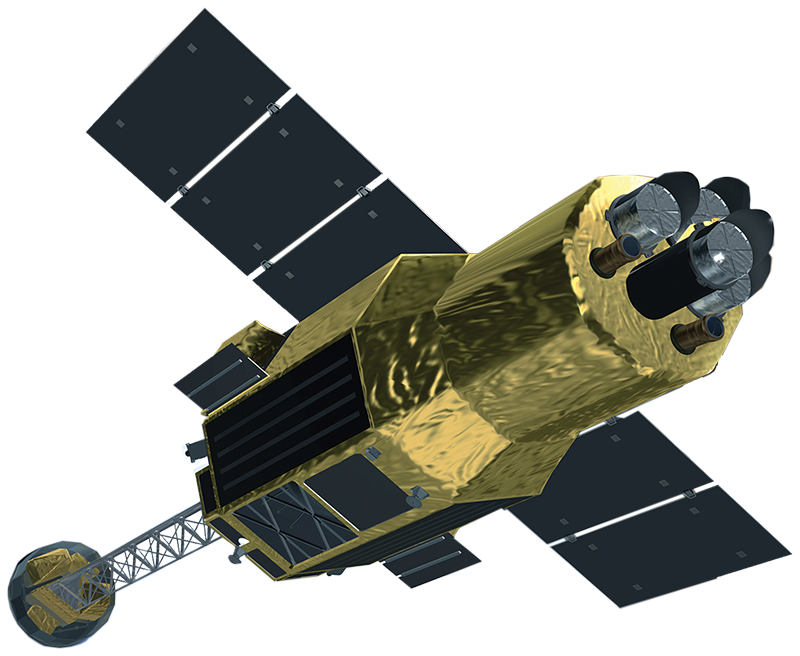
NASA contributed the soft X-ray spectrometer for Japan’s ASTRO-H X-ray astronomy satellite. To carry electricity, but not heat, from a room-temperature power source to a cryogenic superconducting electromagnet aboard the satellite, Energy to Power Solutions developed a set of hybrid, superconductive leads. The electromagnet is used to cool the instrument’s detectors to near absolute zero, making them ultrasensitive.
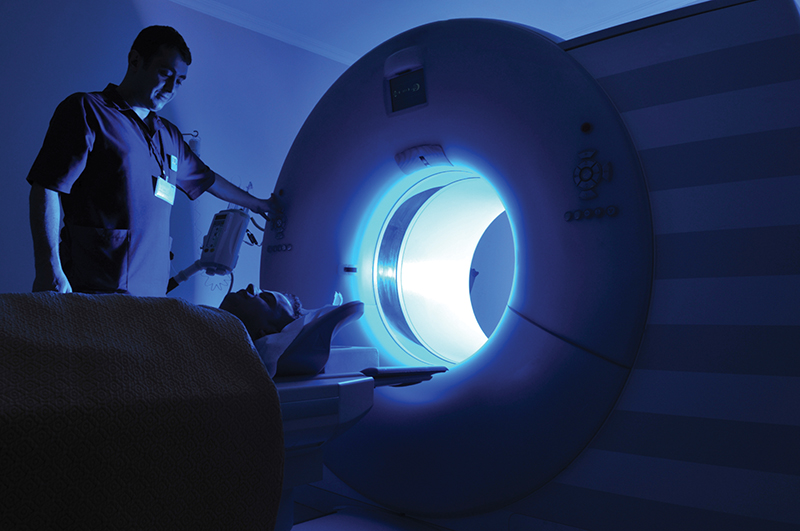
One clear Earth application for the sort of ultra-low-heat superconductive leads that Energy to Power Solutions developed for the ASTRO-H satellite is in magnetic resonance imaging machines, which are also powered with cryogenic superconductors.