
Ruggedized Spectrometers Are Built for Tough Jobs
NASA Technology
Curiosity is the undisputed hot rod of planetary rovers. Nine-and-a-half feet long, nearly 2,000 pounds, and powered by a thermo-nuclear power generator under its “hood,” Curiosity was built to travel far and handle extreme temperature fluctuations. What’s more, its instruments—radiation and gas detectors, imaging cameras, and mineral identifiers, to mention a few—are the most advanced that have ever scoured the Red Planet in hopes of answering that long-pondered question: Was Mars once capable of supporting life?
Another powerful instrument, and the one with perhaps the most visual flair, is the Chemistry and Camera system, otherwise known as ChemCam. It uses what’s called laser-induced breakdown spectroscopy (LIBS) to analyze the elemental composition of materials on the Martian surface. What makes the instrument especially remarkable is its ability to take measurements from as far away as 23 feet and also remove dust from the sample before testing.
ChemCam works by first homing in on a visual target with its mast camera, which includes what’s called a Remote Micro Imager. Then, from its mast, a laser beam potent enough to outshine a million light bulbs (10 megawatts per square millimeter) is fired onto a pinhole-sized area. While the pulse lasts only about five-billionths of a second, it’s enough time for the affected material to reach about 25,000 °F and create plasma, emitting a very bright flash of light and generating a shock wave that ablates the surface part of the material, which enters the plasma. The light emitted by this material is collected by a telescope and travels through fiber-optic cables to the belly of the rover, where a series of dichroic mirrors separates the incoming photons into three wavelength bands to be processed by three different spectrometers, which measure their varying wavelengths and intensities.
Because each element emits specific wavelengths of light, the spectrometer can determine a sample’s elemental makeup. Such information can be used to gain more insight into the planet’s early development, and it also provides clues about which toxins to guard against in preparation for a future manned mission.
Technology Transfer
Roger Wiens, a planetary scientist out of Los Alamos National Laboratory, serves as principal investigator for the ChemCam, which is a joint venture between various US and French organizations. As the person responsible for its final delivery to the Jet Propulsion Laboratory (JPL), the center overseeing the rover’s development, Wiens dedicated the better part of the 2000s making sure the instrument could first handle the rough trip to Mars and, afterward, operate amid the planet’s severe conditions. He wrote about the ordeal in his 2013 book, Red Rover. “Working on this Mars mission was a childhood dream come true,” he says.
But Wiens had some very adult decisions to make, and one of them was selecting a compact spectrometer—the device that measures the wavelengths and intensities of light emitted by a sample material—that was up to the task. It so happened that he had been working with such a device developed by Dunedin, Florida-based Ocean Optics. Founded in 1989, the company had developed the world’s first miniaturized spectrometer, revolutionizing the field. Its optical instruments were being used for a myriad of applications, from determining crop health through foliage color analysis to verifying nutritional content in food commodities.
In the early 2000s, while the ChemCam instrument was still in the proposal stage, Wiens came to an agreement with Ocean Optics to work with his team to make the spectrometer space-ready. In 2004, when the instrument was officially chosen for the mission, the collaboration continued. The company’s high-resolution spectrometer was used as the inspiration and springboard design, but its evolution would ready it for some of the most challenging environments imaginable.
Like most instruments used in space or on other planetary bodies, the spectrometer needed to be protected against the ravages of temperature, vibration, radiation, and shock. On Mars, electronics would need to be qualified for temperatures as low as -65 °F and as high as 155 °F. They also had to survive the intense vibration that would occur particularly during launch from Earth. “Imagine duct-taping yourself tightly to an unbalanced washing machine for eight minutes,” says Dave Landis, who at the time was a vice president for Ocean Optics and a key designer for the project. “It’s that intense.” Then, of course, there’s the radiation to contend with, as the instruments would see radiation exposure both during travel and on the surface of Mars.
The first step, explains Wiens, was putting the commercial spectrometer through an initial “shake and bake” test, referring to the experiments done in evaluating the unit’s temperature and vibration resistance. At the onset, the device’s aluminum casing was replaced with titanium, which, unlike the former, expands and contracts less with temperature fluctuations. But tests showed the optics also needed to be made less temperature-sensitive to avoid defocusing of the spectrometers. Landis, who worked on all aspects of the project, says, “You pick materials that can handle the temperature extremes of space—materials with well-matched thermal coefficients.” He adds that afterward, the reconfigured parts went through 2,000 temperature cycles, replicating the passage of 1,000 days on Mars, to make sure everything worked continuously at a high level.
And following the shake test, it was discovered that some of the mounting systems for the optics, which direct the photons into the electronics for processing, needed redesigning. “If those pieces move during transit, you can corrupt the data from the instrument to the point where it’s not useful,” says Landis. “So you pick glues and epoxies that are going to survive the shock loads because you’ve got glass bonded to metal.”
Wiens also notes that the device’s optical throughput, its ability to receive light, was optimized. “We made specialized mirrors to optimize the slit configuration and changed the detector.”
All that work, which also included hardening various electronics against radiation, was completed over a span of several years at several locations, up until ChemCam was delivered to JPL in 2010. As the world witnessed, the LIBS spectrometer launched with the rover in November 2011, and since landing the following August, the instrument has identified more than 140,000 samples. Among its most important discoveries was the detection of calcium, which led to the discovery of gypsum and bassanite, minerals formed as a byproduct of calcium sulfate and water: a key building block for life.
Benefits
Ocean Optics’ spectrometers have helped uncover Mars’ secrets, but the work the company did with NASA to ready their devices for that otherworldly mission has also paid dividends here on Earth. That’s because some of the improvements made to ready the spectrometer for space have been incorporated into Ocean Optics’ newer spectrometer models.
One of the big changes they’ve made is to decouple the spectrometer detector from the electronics. Though not housed externally like in the ChemCam, just separating these two components within the spectrometer housing improves the long-term wavelength stability. “It’s incredible to think that we can achieve mere picometer-level drift over a period of hours of operation with our EMBED spectrometer,” says David Creasey, the firm’s vice president of sales and marketing. “That kind of performance is paramount for some of our OEM [original equipment manufacturer] customers.”
The measures the agency took to protect the spectrometers from extreme temperature swings have also inspired improvements in optics mounting methods, improving performance when exposed to a dramatic range of warm and cold environments. “We have spectrometers that are used in research applications from Antarctica to volcanic peaks and the Amazon,” Creasey says. “Our units need to be robust enough to make it there, and thermally stable enough to take high-quality measurements once in place.”
Having sold more than 250,000 spectrometers, the company is the most prolific manufacturer of such devices in the world. Its history of collaboration with the space agency, says Creasey, only adds to its appeal for organizations wanting a reliable device. “With each new spectrometer line we launch, we integrate our experience with scientific leaders like NASA and our OEM customers to push the boundaries of miniature spectroscopy further. We make each other better, and that is truly what science is all about.”
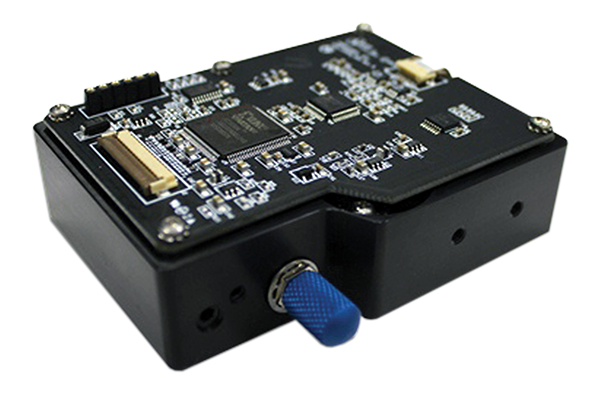
Ocean Optics collaborated with NASA to redesign its miniaturized spectrometers for use in space and has since transferred those technologies to some of its commercial product lines, which are now more resistant to the effects of shock and vibration and are more protected from extreme temperature swings. Pictured here is the company’s EMBED spectrometer, which offers those enhancements.

One of Ocean Optics’ NASA-improved spectrometers is attached to an all-terrain vehicle, which is utilizing the instrument for analyzing upwelling and downwelling types of radiation in the desert. The temperature- and shock-resistant properties of the spectrometer prove vital in such a rugged environment.